
The genesis of life
Life's origins
Did the egg or the chicken come first? This question is often pondered regarding life’s origin and how biological systems came into play. How did chemistry move to biology to support life? And how have we evolved into such complex organisms? The ingredients, conditions and thermodynamically favoured reactions hold the answer, but understanding the inner workings of life’s beginnings poses a challenge for us scientists. Under an empirical approach, how can we address these questions if these events occurred 3.7 billion years ago?
The early atmosphere of the Earth
To approach these questions, it is relevant to understand the atmospheric contents of the primordial Earth. With a lack of oxygen, the predominant make-up included C02, NH3 and H2, creating a reducing environment for the drive of chemical reactions. When the earth cooled, and the atmosphere underwent condensation, pools of chemicals were made - this is known as “primordial soup”. It is thought that reactants could collide from this “soup” to synthesise nucleotides by forming nitrogenous bases and bonds, such as glycosidic or hydrogen bonds. Such nucleotide monomers were perhaps polymerised to create long chains for nucleic acid synthesis, that is, RNA, via this abiotic synthesis. Thus, if we have nucleic acids, genetic information could have been stored and passed later down the line, allowing for our eventual evolution.
Conditions for nucleic acid synthesis
The environment supported the formation of monomers for said polymerisation. For example, hydrothermal vents could have provided the reducing power via protons, allowing for the protonation of structures and providing the free energy for bond formation.
Biology, of course, relies on protons for the proton gradient in ATP synthesis at the mitochondrial membrane and, in general, acid-base catalysis in enzymatic reactions. Therefore, it is safe to say protons played a vital role in life’s emergence. The eventual formation of structures by protonation and deprotonation provides the enzymatic theory of life’s origins. That is, some self-catalytic ability for replication in a closed system and the evolution of complex biological units. This is the “RNA World” theory, which will be discussed later.
Another theory is wet and dry cycling at the edge of hydrothermal pools. This theory Is provided by David Deamer, who suggests that nucleic acid monomers placed in acidic (pH 3) and hot (70-90 degrees Celsius) pools could undergo condensation reactions for ester bond formation. It highlights the need for low water activity and a “kinetic trap” in which the condensation reaction rate exceeds the hydrolysation rate. The heat of the pool provides a high activation energy for the localised generation of polymers without the need for a membrane-like compartment.
But even if this was possible and nucleic acids could be synthesised, how could we “keep them safe”? This issue is addressed by the theory of "protocells" formed from fatty acid vesicles. Jack Szostak suggests phase transition (that is pH decrease) allowed for the construction of bilayer membranes from fatty acid monomers, which is homologous to what we see now in modern cells. The fatty acids in these vesicles have the ability to “flip-flop” to allow for the exchange of nutrients or nucleotides in and out of the vesicles. It is suggested that clay encapsulated nucleotide monomers were brought into the protocell by this flip-flop action. Vesicles could grow by competing with surrounding smaller vesicles. Larger vesicles are thought to be those harbouring long polyanionic molecules - that is RNA - which creates immense osmotic pressure pushing outward on the protocell for absorption of smaller vesicles. This represents the Darwinian “survival of the fittest” theory in which cells with more RNA are favoured for survival.
The RNA World Hypothesis
DNA is often seen as the “Saint” of all things biology, given its ability to store and pass genetic information to mRNA and then mRNA can use this information to synthesise polypeptides. This is the central dogma of course. However, the RNA world hypothesis suggests that RNA arose first due to its ability to form catalytic 3D structures and store genetic information that could have allowed for further synthesis of DNA. This makes sense when you think about how the primer for DNA replication is formed out of RNA. If RNA did not come first, how could DNA replication be possible? Many other scenarios suggest RNA evolution preceded that of DNA.
So, if RNA arose as a simple polymer, its ability to form 3D structures could have allowed ribozymes (RNA with enzymatic function) within these protocells. Ribozymes, such as RNA ligase and polymerase, could have allowed for self-replication, and then mutation in primary structure could have allowed evolution to occur. If we have a catalyst, in a closed system, with nutrient exchange, then why would life’s formation not be possible?
But how can we show that RNA can arise in this way? The answer to this is SELEX - selective evolution of ligands by exponential enrichment (5). This system was developed by Jack Szostak, who wanted to show the evolution of complex RNA, ribozymes in a test tube was possible. A pool of random, fragmented RNA molecules can be added to a chamber and run through a column with beads. These beads harbour some sequence or attraction to the RNA molecules the column is selecting for. Those that attach can be eluted, and those that do not can be disregarded. The bound RNA can be rerun through SELEX, and the conditions in the column can be more specific in that only the most complementary RNAs bind. This allowed for the development of RNA ligase and RNA polymerase - thus, self-replication of RNA is possible. SELEX helps us understand how the evolution of RNA in the primordial Earth could have been possible.
This is also established by meteorites, such as carbon chondrites that burnt up in the earth’s atmosphere encapsulating the organic material in the centre. Chondrites found in Antarctica have been found to contain 80+ amino acids (some of which are not compatible with life). These chondrites also included nucleobases. So, if such monomers can be synthesised in a hostile environment in outer space/in our atmosphere, then the theory of abiotic synthesis is supported.
Furthermore, it is relevant to address the abiotic synthesis of amino acids since the evolution of catalytic RNA could have some complementarity for polypeptide synthesis. Miller and Urey (1953) set up a simple experiment containing gas representing the early primordial earth (Methane, hydrogen, ammonia, water). They used a conduction rod to provide the electrical discharge (meant to simulate lightning or volcanic eruption) to the gases and then condensed them. The water in the other chamber turned pink/brown. Following chromatography, they identified amino acids in the mixture. These simple manipulations could have been homologous to early life.
Conclusion
The abiotic synthesis of nucleotides and amino acids for their later polymerisation would support the theories that address chemistry moving toward biological life. Protocells containing such polymers could have been selected based on their “fitness” and these could have mutated to allow for the evolution of catalytic RNA. The experiments mentioned represent a small fragment of those carried out to answer the questions of life’s origins. The evidence provides a firm ground for the emergence of life to the complexity of what we know today.
Written by Holly Kitley
Project Gallery
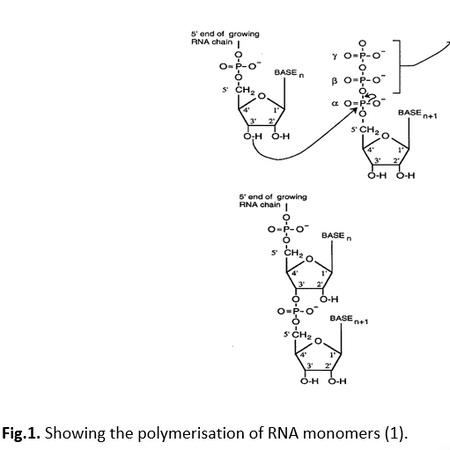
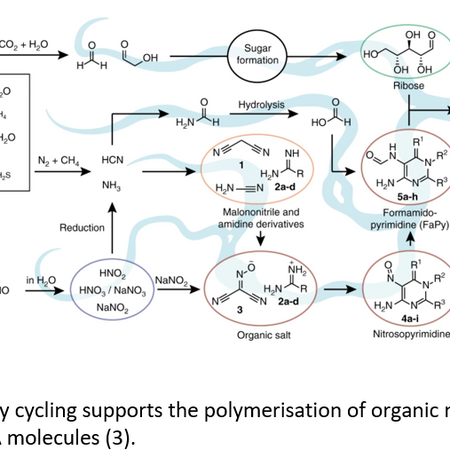
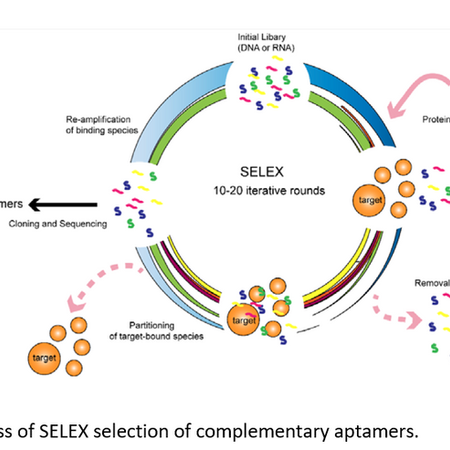

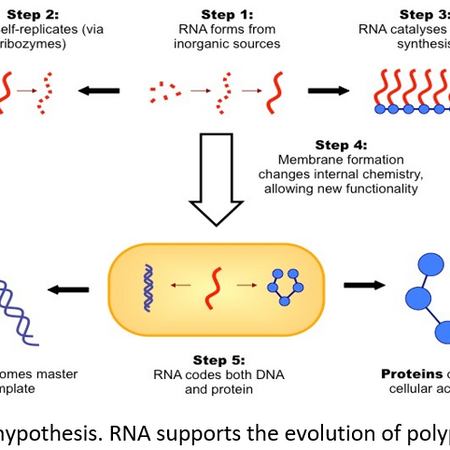
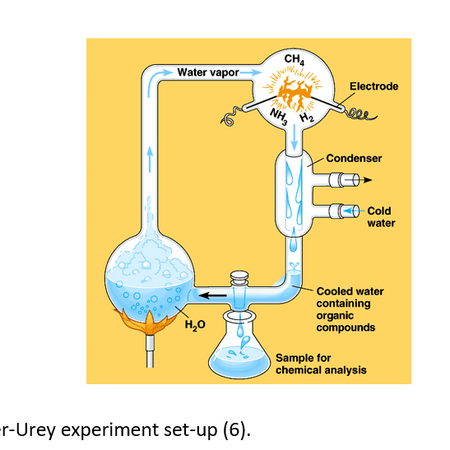